THE PHYSICAL CELL:
The cell - strange crucible, battery and computer ...
Apologies are necessary where in the rest of this site
imaginative construction (or sheer ignorance) in one way or another offends or is felt inappropriate. Mea culpa every
inch and, ultimately, every detail might be wrong, but it is a gedanken experiment really only attempting
a proof of principle, i.e., that living systems are what they are, can only be what they are, because
they are quantum systems. Hyper-evolved quantum systems at that.
If that much is recognised - and it
seems it will be very soon - it will bring science to a very important pass with respect to the mythmaking of man that is
embodied in the belief system that is science and that which is embodied in the various belief systems that in man's
culture become elaborated as 'faith' of one sort or another, some going on to achieve a state of organisation
sufficient to become recognised faith systems. In essence, the quantum approach requires that science prepare itself
to deal head-on with questions around intelligent design - and then provides some means to do so. Paradoxically,
however, its every evidence also works to favour those who would make construction in ID terms.
It needs
to be recognised perhaps that whilst both science as faith built on past experience and belief as faith built on some totality
of experience, both - the former only more recently - question the means of our existence, search to find every step and event
that led to our coming into being, seek pattern and reason in the apparent vagiaries of nature's play and, therein, to
understand the role of man in all this. The latter, however, has always recognised the necessarily greater power
that resides in process, rather than celebrating the created, and thus finite, object. The dimensional landscape
of those that seek to interpret the nature of the possible complex realities that underpin our existence, whilst still 'rationally'
acknowledging the cosmos as being of a higher dimensional complexity than one's own local reality, seem somehow
assured of a richer internal life, at the very least.
Quantum systems self-organisation cannot be anything
but 'intelligent', in so far as the componental relations need be mediated by the passaging of quantised wave energies
and processes will therefore, at times, show quantum sensitivity and be founded in their energetic relations by quantum steps.
Some illustration of what is meant by this is given below using the data for L-amino acid specific rotation,
i.e., optical activity in terms of bending the path of plane polarised light. As can be seen from the radar plot
below, the variety of the L-form amino acid array, when organised by reference to the specific rotation, throws
up a comfortably stepped - 5 degrees, average? - arena for the rotational passaging of light - and proline acquires new
significance in terms of our understanding of living systems as exemplar for computing with light.
It
would seem inevitable then that relations with those that work from the premise of intelligent design will, in the quantum
perspective that science is now developing, need to work towards recognising the common ground that is their interest,
i.e., the nature of reality, at least, whilst then agreeing to differ with respect to any common cause. Science
today, anyway, has accepted the 'uncaused' cause and by this may fairly defend the approach.
The intent of this preamble is to ensure that an 'interpretive' relativity obtains in this site, i.e., what the
arrangements of nature have to say about the nature of nature is for each man himself to interpret and decide, i.e.,
'God' is in the eye of the beholder.
Most immediately what follows is indication of the types of deconstructive
thinking and reconstructive models that we might hope to use in remodelling our understanding of cell structures and dynamics.
Strictly speaking this and the two following pages of the
site should be couched in terms of bioelectromagnetics theory and research - effectively acknowledging the atomic base in
proposing the whole cell as an oscillatory system and their communication or coherence being maintained by light (as biophotons)
- but given that this vast literature has been missed even by some of the leading lights and journals of our current science
there can be no hope of here making good such a shortfall and illustration will, largely, be most general and at the macroscopic
scale, merely to give indication of some of the principles that might be used to make the transition from the phenomenology
of biological analysis to its more quantum base. It will be necessary, however, in the next section, which has to do
with the working and informational significance of the cellular outputs that are DNA, RNA and proteins, to take the quantum
approach.
Organic foundations in a new light
It has to be
borne in mind that our modelling in future must acknowledge the changing status of organic or carbon chemistry.
Not only do we find indication by analogue that we have to remodel our thinking in the light of the buckyball research
of Zeilinger et al., where the quantum state is found to be maintained through to 1,000 K before wholesale photonic collapse,
but also buckytube research tells us about electron tunnelling within and through the tube wall, thereby entraining
the residual ion population dynamic. These researches provide us with early analogues for understanding
the real physics of membranes as vast sheets of superconducting 5- and 6-node cyclic carbon, forming vesicular cells or,
in pure hexagonal array, as 6-node cyclic carbon rolled into tubes.
Even when we come to understanding
the classical and quintissential system of biology that is a protein, we today have to take into account the phenomena
of electron and proton tunnelling and the uses made of quantum 'noise', coherence and annealing. Even the
literal and physical core of life science, the DNA molecule, is now subject to interpretation in the light of quantum and
complex systems interpretation as founded in the new non-linear mathematics of chaos theory and fractal geometry and evidenced
in such publications as, e.g., Non-Linear Physics of DNA (Yakushevich, 2nd. ed., 2004). Further,
beyond these considerations, there is also need to accommodate the wider transition of vision currently underway with respect
to the role of carbon as the natural material to replace silicon in terms of quantum computing development. Thus, it
seems, classical understanding ends and a new understanding emerges at the level of working at which life itself
must have begun.
|
Classical cell membrane modelling |
Cell membranes as Russian
doll-packed buckyballs?
Traditional biological modelling of the cell membrane (above right) says little
about the cohesive lateral forces that operate and enable this lipid effected subdivision of space. Whilst the lipid 'head'
groups and membrane-associated protein arrays receive much attention in characterising cell types, one has to suspect
that structurally and functionally it is the long carbohydrate 'tails' and their lateral interaction, in
quantum terms, that act to form the real 'wall'.
Modelling from first principles
requires that the system being mapped is worked to the extremes, i.e., beyond the limit of the parameters that define the
system itself, here consisting of linear carbon chains and associated protons:
(i) In the case of excluding
the hydrogen, we immediately see that the carbon-carbon bonding then required naturally falls to the pentagon and hexagon
arrangements that allow for the curvature of a Buckysheet to be sufficient to form a sphere or Buckyball.
Buckyballs, as we all know, have interesting properties, not least the fact of them being superconducting below 3 K
and, as was shown recently by Anton Zeilinger, maintaining their quantum state through to 1,000 K. Against this
pure carbon form we must then wonder at the effect on those quantum properties when the protons are reintroduced and interpolate
the bonding pattern, thus creating quasi-buckyballs. Does this make these quasi-buckyballs some sort
of proton capture or capacitor system?
The living cell membrane even further extends the quantum requirements
for modelling in so far as, given the number of carbons in the lipid tail, we effectively have that number of quasi-buckyballs
in Russian doll-type nested array. The endohedral limits of pure bucky structures - balls or tubes - are actively being researched,
but there is a long way to go from modelling a few hundred carbons in the plane - where we are now - to mapping
the millions in even the smallest cell membrane, let alone the effects of proton doping and Russian doll packing.
The proton traffic within this arrangement would of course not be limited to the plane and so it becomes a quasi-quasi
buckyball, an object still to be explored by fundamental physics.
(ii) Modelling in the other
extreme we remove the carbon from our model and we are left with a 'membrane' of free hydrogen which itself
would form an ionic net by electron sharing, free protons then constituting the nodes of this essentially photon-based
electronic and ionic net. Effectively, physically, it is a plasma, the fourth state of matter of which again, as
yet, we know little, even on the cosmological scale we are used to exploring, let alone at the nano-scale we are
now entered upon.
Given such considerations as to the lateral transport patterns in the plane of the membrane,
we can perhaps then take the larger scale phenomenon we are used to - the Faraday cage effect - and realise the cell interior
to be a highly protected environment although, importantly, still subject to external influence in some respects. Certainly
membranes can be seen as acting to enclose and isolate sub-states of the larger dynamic. In this much at least
membrane organisation acts to change the time dynamics of reactions, either delaying or speeding the 'natural'
physical runout of the reaction, much as proteins, as enzymes, can themselves change the rate of reaction.
Further indication of the power of membrane
enclosure becomes evidenced when we look at the smaller membrane bound vesicles that are held within the cytoplasm of the
cell - such as the peroxisomes, lysosomes and blebbing vesicles of the Golgi apparatus and endoplasmic reticulum - in so far
as these have a twofold function controlling both the reaction rate of the contents and then shielding the point process and
products from interference. The endoplasmic reticulum and the Golgi apparatus, by their flattening and close apposition
of surfaces might be expected to be found to be conformations that modulate such quantum properties. The control
of reaction rates is extrapolated from the same caging effect being used at the scale of single molecules, e.g., hemi-carcerands,
and it is surely a reasonable expectation that the phenomenon of protein coating of membraneous vesicles, e.g., clathrins
or nuclear lamins, acts to modulate and amplify this functionality.
It is interesting to compare the mainstream lipid bilayer
modelling and this more quantum interpretation. First the classical description via Wikipedia (with amendments)
on Membrane Potential:
'Classically, in electrical terminology, the plasma membrane functions as a combined
resistor and capacitor. Resistance arises from the fact that the membrane impedes the movement of charges across it
and capacitance arises from the fact that the lipid bilayer is so thin that an accumulation of charged particles on one side
gives rise to an electrical force that pulls oppositely-charged particles toward the other side.
The capacitance
of the membrane is relatively unaffected by the molecules that are embedded in it, so it has a more or less invariant value
estimated at about 2 µF/cm2 (the total capacitance of a patch is proportional to its area).
The conductance of a pure lipid bilayer is so low, on the other hand, that in biological situations it is always dominated
by the conductance of alternative pathways provided by embedded molecules. Thus the capacitance of the membrane is more
or less fixed, but the resistance is highly variable.
The thickness of a plasma membrane is estimated to be about 7-8 nanometers. Because the membrane
is so thin, it does not take a very large transmembrane voltage to create a strong electric field within it. Typical
membrane potentials in animal cells are on the order of 100 mV, i.e., one tenth of a volt, but calculations show that this
generates an electric field close to the maximum that the membrane can sustain - it has been calculated that a voltage difference
much larger than 200 mV could cause dielectric breakdown, that is, arcing across the membrane.'
The quantum descriptive agrees with classical observation,
but then extends to give better grounds to the real energetic dynamics of the phenomenon, e.g., impeding charge movement
can be effected by the field generated by charge movement, i.e., within the buckyball layering quantum flow allows for
the switching of protons and electrons such that their lateral or tangential flows and generated fields then prevent
the free passage of charged ions.
'The capacitance of the membrane' 'has a more or less invariant
value estimated at about 2 µF/cm2' accords with the idea that the main element of this subsists in the
invariant element of the membrane, the number of buckyball planes. The measure given is still enough to kick around
1.2 x 1018 electrons or 6.4 x 1014 protons.
'Capacitance arises from the fact that the
lipid bilayer is so thin that an accumulation of charged particles on one side gives rise to an electrical force that pulls
oppositely-charged particles toward the other side.' Given that the capacitance at any point will depend on
the membrane flows and, therefore, be variable at any particular point, external or internal disequilibria can act through
the membrane to bias the flows, effectively creating a sort of flux pinning and inducing equal and opposite change in
the connected systems.
'The conductance of a pure lipid bilayer is so low, on the other hand, that in biological
situations it is always dominated by the conductance of alternative pathways provided by embedded molecules.' We
can see here then that the sheer noise of the membrane and the stochastic nature of transport across such, both then in undirected
activity, will naturally be superceded in efficiency by membrane inserted proteins devoted to the passaging of particular
ions and which, if able to make use of the membrane noise, i.e., free quanta of various energies, can then become pump type
channels that can then work counter to the physical principles, e.g., transport against a concentration gradient.
Thus the capacitance of the membrane is more or less fixed, but the resistance is
highly variable.
'The thickness of
a plasma membrane is estimated to be about 7-8 nanometres and because the membrane is so thin, it does not take a very large
transmembrane voltage to create a strong electric field within it. Typical membrane potentials in animal cells are on
the order of 100 mV, i.e., one tenth of a volt, but calculations show that this generates an electric field close to the maximum
that the membrane can sustain - it has been calculated that a voltage difference much larger than 200 mV could cause dielectric
breakdown, that is, arcing across the membrane.'
The figure of dielectric breakdown
at 200 mV which Wikipedia suggests needs to be born in mind when we come to look at the electrolytic arrangement
of the cell and sub-spaces therein.
The cell behaviour around cell division
also tells us something of the physical nature of the cell interior and the capacity to act as an electrorheological fluid,
whereby it is via a calcium cascade that detachment of the cell matrix from the plasma membrane is realised. It is a
cascade and, therefore, has ongoing effects at ever finer scales. Generally, current changes within such a gel act to
alter the geometry of the gel components and, therein, a variety of connectivities. In living systems this might have
to do primarily with altering the relative phase states of water, the fundamental energetic metric and network for living
systems processing and information transfer, with concomitant significance therein for the contained proteins and free ions.
Releasing the cell matrix from the variable geometry imposed by various membrane proteins and their connections sets
it free to self-adjust against internal physical changes, so producing the distinctly non-'minimal' structure that
is the 'rounded-up' cell entering division. Indeed, much research today is focussed around understanding
the geometric principles of the interphase state of cells in order to achieve the induction and commitment of stem
cells to a particular cell lineage, e.g., bone.
Such an electrorheological principle for self-organisation
would also then assist in explaining how the plane of the metaphase plate becomes set at 90 degrees to the axis generated
between the centrosomes in formation of the mitotic spindle, but we first need to review these and other biological objects
or sub-systems, in terms of their physics, before this becomes clear.
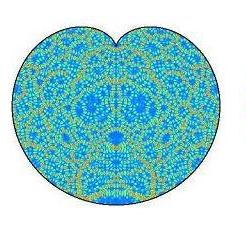
Bounded dynamics
Over and above all considerations is the principle role of the membrane, the partitioning of space, which for all levels
of systems sets a necessary bias to the expected outcomes of free process. Given that the bounded system must operate
at a higher energy density than the environment, the membrane, in and of itself, acts to counter the 'natural' physical
dynamic that would work to homogenise the distribution whilst then also acting to constrain the dynamics of the
contained system. Where a membrane encloses a generate system or core, the bounded dissipative dynamics of the
output become folded back on themselves, this feedback presenting as chaos or noise until focus is realised back at the
source, where all feeds eventually become focussed. Think of a caustic curve in three dimensions, otherwise known
as a cardioid. This topological understanding is encouraged by the geometry of the metaphase state that persists in
the human ovum until collapsed by the calcium cascade initiated by fertilisation.
|
(1) Egg spindle and metaphase plate (2) first polar body spindle and plate (3) Perivitelline space |
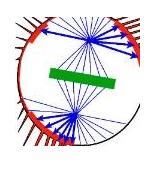
The diagram (far left) of the egg in
the electrorheological and glass-like state of suspended animation that is the meiotic second metaphase,
shows how the external presence of the polar body makes a caustic of the egg interior and, then, how this
is amplified by the suspension of the spindle via 'retractor' microtubules attached to old cell-cell adhesion
sites at their membrane ends (above) and focussed in the pair of centrioles and their associated pericentriolar material,
which together form the centrosomes or asters, at their 'nuclear' end.
Further, the diagram highlights
the 90 degree rotation of the egg and polar body spindles and metaphase plates suggesting these macroscale systems are
entangled.
Within the developing egg the subsequent planes of division and their relative 90
degree displacements after each division event again imply that an electrorheological or electrophoretic analysis
would be most useful and in crudest terms results in a three step walk between recurrences of cell state in strictly
physical terms.
|
Cardioid chaos (left) and helical ordering emerging out of higher frequencies |
Much is being made here of the idea of 'the boundary' as it is of singular
and fundamental importance - being in some respects a 'constraint' - in our understanding of all systems. Whilst
important for our scientific modelling of living systems it gives us also direct connection with mathematical theory in so
far as it has purest conceptual expression in the Gaussian Plane which arises in any boundary mapping, the invisible mid-point
of passage where the boundary being breached might act to allow or oppose transit.
As a brief illustration
of the importance for mathematics as well as for living systems modelling, the cardioid contained chaos pictured
earlier and above (left) is taken from the web pages of Jens Marklof (Bristol University, UK) and was offered
as an open question for answer. The explanatory needs be given almost verbatim:
'[Above] is the image of the intensity distribution of two quantum wave functions (eigenstates)
of an electron confined to the cardioid. (Blue corresponds to low, Yellow to medium and Red to high intensity,
courtesy of A. Bäcker, TU Dresden). One of the central theorems in quantum chaos (Shnirelman's quantum
ergodicity theorem) says that, if the underlying classical dynamics is chaotic, then almost all eigenstates look as the one
above on the left, in that they are uniformly distributed over the whole domain. Localised eigenstates
such as the one above right are very rare and it is not clear whether these still exist at very high energies, i.e., small
wavelengths.'
It is here that quantum life science and the fractal geometry of chaos will find a
meeting ground, as we can all see that the apparently chaotic state of a bounded set of frequencies has embedded within it
a high frequency and near helically-ordered core. The emergence of helically ordered DNA within the cell, perpendicular
to the axis of the internal caustic induced by the presence of the nucleus, for living systems at least, tells us that 'Localised
eigenstates such as the one above right do still exist at very high energies, i.e., small wavelengths.'
Indeed, this mathematical construction suggests that it is the high energy of such states that requires fractal structuring
of the embedding environment so as to be able to lay off these high frequency energies to more massive, larger, sub-harmonic
or lower frequency systems. Further, it is suggested, that dissolution of the high energy, small wavelength state
in the helical core - in other words, the 'unwinding' of DNA, i.e., going into working or protein producing mode -
will change the relative ordering of states within the chaotic array, thereby effecting change through the whole bounded set,
in the cell this being the whole of the cytoplasmic array.
Direct enquiry as to this possible identity solicited
no response, so there will be some utility for the mathematicians if quantum life science could eventually complete
the picture with details from the exemplar that is the living cell.
Perhaps worth noting here that the living cell will
always be taken as exemplar as it has ontological priority over all other elaborations.
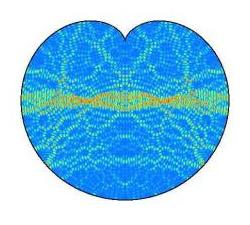
The cytoskeleton
Whilst the membrane bound systems of the cell need review in the light of
the above considerations, the other main dynamic component of the cell matrix is the cytoskeleton and modelling here
has already moved to a more physical focus and theoretical extension in the work of Hammeroff and Penrose.
In essence their work has proposed the microtubule processing of tunnelling electrons to offer a means to our understanding
of consciousness. It will be seen that in fact we do better to understand these long tubes as analogous to the myelin
sheathing that protects signal transport in the larger organism, i.e., the axonal structures, and certainly that functionality
would allow for the emergence of such a thing as kinetic learning, co-ordination and memory in the cell body, just as
is achieved in all the simplest organisms, but we have to suspect that the 'seat of consciousness', if there is in
fact any such thing, itself lies elsewhere.
The cytoskeleton operates with three levels of scale for
the components. At the finest scale actin and myosin form a fine meshwork throughout the volume of the cell and intermediate
filaments constitute a mid-scale scaffold, together with the larger components of the cytoskeleton, the microtubules,
then forming a three level structured net. These networks can extend from the cell membrane, through the cytoplasm,
into the nucleus and then to the chromosomal array, but of course, at various phases in the cycle, one or other of these state
critical connective systems must undergo collapse in order to allow transformation or change of state.
At a simplistic level we can model microtubules as rolled Buckysheets and so might expect them, as a result of the
valency electrons that remain free in this carbon bonding pattern, to offer some similarly extraordinary quantum properties.
Part of the data used earlier in plotting the superconduction properties of atomic mixtures does include the instance of the
single component superconductor and Buckytubes fall into this category. Whilst Buckyballs are superconducting up to
3 K, Buckytubes are in fact superconducting up to 15 K.
Whilst superconduction is the phenomenon of
interest at the cold end of things, Zeilinger et al., have produced a number of experiments showing that Buckyballs retain
their quantum nature, i.e., produce diffraction effects, through to 1000 K, white body temperature, at which point quantum
leakage becomes wholesale. This alone should have given classical biology pause for thought as to the working
frame they seek to understand. Had this been written earlier the proposal of microtubule superconduction
would mainly have had to refer to the work of Hammeroff and Penrose, but recently reported research exploring the physics
of black holes offers another path to confirming such thinking and is of such significance as to be worth reporting directly:
'An artificial "black hole"
designed to capture wayward atoms could pave the way for an atom trap that will yield previously unknown states of matter.
A team led by Lene Hau of Harvard University has mimicked the death spiral of matter falling into a cosmic black hole.
By applying a voltage across a carbon nanotube - a rolled-up sheet of carbon atoms - the team can generate a powerful electric
field. This tugs at nearby [] atoms which have been chilled to a fraction of a degree above absolute zero: a positive
charge on the surface of the nanotubes attracts the [] atoms' electrons, while the positively charged nucleus is repelled.
This polarisation causes the atoms to spiral towards the nanotube, speeding them up until the atoms circle it in just
a few trillionths of a second. Eventually each atom's outermost electron detaches and enters the nanotube through
a process called quantum tunnelling. The positively charged [] ion that it leaves behind is repelled by the positively
charged nanotube and slingshots away.
Modification to this set-up could produce a trap capable of keeping a cloud
of cold atoms spinning around the nanotube.' (New Scientist, 17 April 2010, p 15). (The research was based
on behaviours with Rubidium atoms, but of course we can extend it to any atom for the purposes of modelling here).
There are a number of models we might use to illustrate the above and two are shown below, but each in essence
works so as to generate a central vortex filament within the wider trajectory mapping of the vortex-susceptible particle species.
|
Belousov-Zhabotinsky phase portrait |
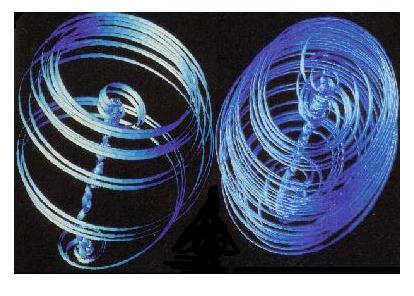
|
Taylor-Couette type modelling for regular (left) and increasingly chaotic flow dynamics (right) |
This research has great significance not only for interpretation
of microtubule functionality in terms of quantum physics, but also all other tubular arrays found in the cell such as the
basal bodies and flagella, the chromosomes perhaps, but most importantly here, in terms of providing a dynamic focus,
the centrioles, two short tubular objects whose walls are threefold tubulin plates arranged like the vanes on a turbine
or the planes of a paddlesteamer wheel and whose duplication and dynamics ordinate and regulate cell processes to do
with nuclear activity, nuclear dissociation and cell duplication.
Given the turbine-vane arrangements that obtain
in terms of microtubule array and the behaviours we now know each tubule might generate in terms of entraining ion
behaviour, it is surely possible to appreciate the forces that then might operate in terms of interpreting this as some
sort of atomically scaled turbine. That the natural bias of alpha and beta tubulin subunits then sets
the larger polarity for the tubule and, therein, sets up the dynamics of ion entrainment around the whole construction, merely
as the result of the charge disequilibrium that can be guaranteed to obtain between the ends of any tubule in any direction
at any time, implies that we have a highly sensitive and perpetual driver for microtubule based ion dynamics.
It
is poetic then that the Lorentzian dynamics that we find underpinning the dynamics here also have expression as the symbol
for infinity.
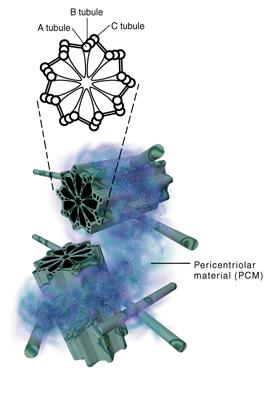
|
Schematic presentation of centriole cross section and in vivo arrangements |
|
Electron micrograph of the centrioles and the electron dense pericentriolar material connecting them |
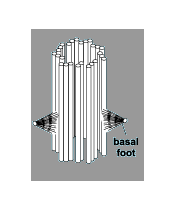
|
Mother centriole - daughter arises at other end to basal 'foot'? |
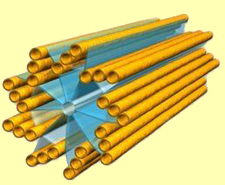
|
The standard daughter centriole |
|
The gamma tubulin in the pericentriolar matrix fixes the microtubule (-) end |
As can be seen from the above illustrations the usual two centrioles (left) have a very characteristic 90 degree arrangement
between them and, in this, the mother and daughter centrioles (above and above right) seem to present two of three
axes. Such a sharp and constant arrangement bespeaks electromagnetic action, just as the Fleming rules work around three
axes of field, force and motion.
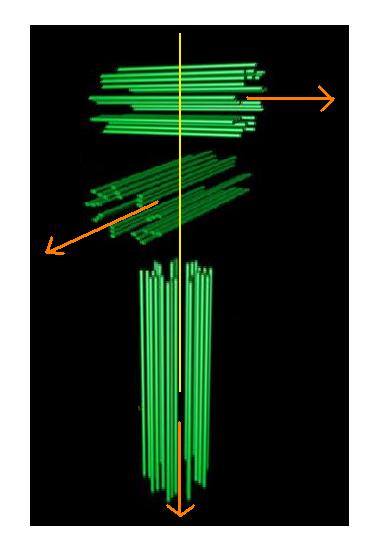
|
The three 'poles' of the centrosome generated by the two centrioles and the microtubule array |
The third axis emerges as a result of the dynamics
of the microtubule array becoming focussed in formation of the division spindle and the movement of the chromosomes
by a sub-set of these microtubules towards the metaphase plate seems to confirm the field/force/motion idea.
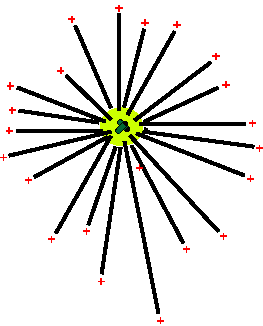
|
The centriole acts as a microtubule source and focus |
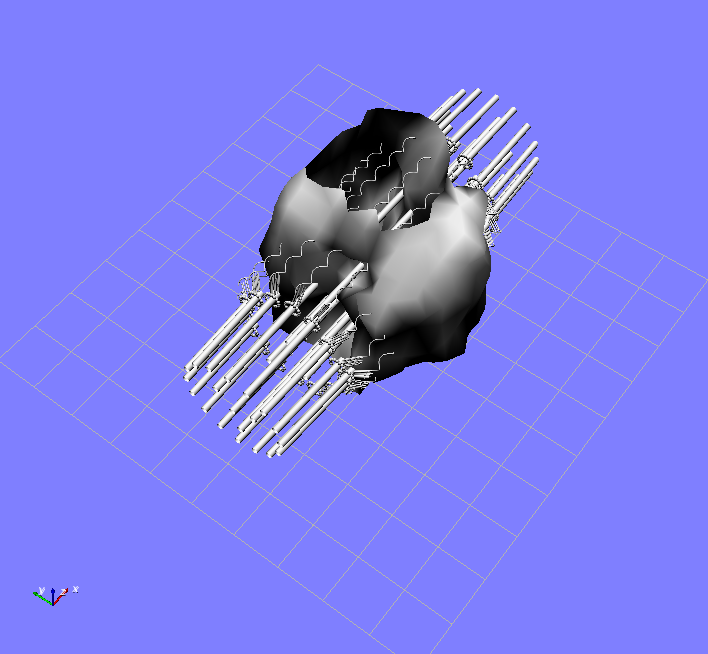
|
The microtubule assisted progression of the chromosomes to the metaphase plate |
It is this physical construction with respect to nanotubes
and their electron tunnelling, superconducting walls and the 'entrained slingshot' - effectively vortical - dynamics
of the parent ions, that then helps explain the more general case that is found whereby nanotubes will effect a
polarisation of the environment by producing a separation of charge. When we use a suitable
illustrative model for this and replace all tubular systems in the cell with a slingshot model we seem to have hope of explaining,
for example, with respect to centrosomes, the accumulation of ions in the pericentriolar material as a result of the slingshot
dynamics running across the centriole pair; with respect to microtubules, their dynamic roles in terms of polarising
the cytoplasmic environment; and, of course, with respect to the chromosomes, when maximally wound, their dynamics of response
to the imposed charge dynamics and their own extension of the principle in their own working.
This takes us from the Lorentzian behaviour
that the centriole and periocentriolar protein connect would suggest for the free ions, to the extended Lorentzian of
the microtubule, in much extended form, inducing the same ionic entrainment. The extended form and polarity of the microtubules then
acts to ensure a disequilibrious separation of charge in the volume of the cell and, we have to suspect, identification of
the centrosome as source and the (+) end of the microtubule as sink.
Given the natural requirement for symmetry
we might therefore expect that the microtubule, rather than at the the source end feeding off entrained ions, at the
sink end acts to induce the formation of a similar arrangement - i.e., a superconducting tubular array - that might then serve
to clear the ion cloud accumulating at the (+) end? We have to suspect that such a structure might either be the centromere
of the classical chromosome or, indeed, more likely, as perhaps seed to that formation, the peri-centromeric arrangements
that are found to obtain for attachment of the microtubule end to the centromere, i.e., the kinetochore suite of proteins?
|
Knockout mutant cells produce multiple daughter centrioles (coloured) |
An interesting possibility is raised by the fact that
when part of the normal functioning of centriole development is knocked out, many daughter centrioles can be formed (above,
right) and these, naturally, come to lie at the equator of the mother centriole. Centriole formation can also be induced by
the application of an electrical current and prompts the realisation that centriole appearance might be taken as signal for
the energetic state of the cell.
The generation of multiple centrioles, as we readily know, will disrupt
what we now regard as the normal polar arrangements of the cell, with concomitant effect for the division products.
Given, however, that the cell can produce such multiple centrioles and 'normal' operation requires only two, it may
well be that the spindle microtubule array has evolved from such additional centrioles and their triplet structure being 'decoupled',
wherein the enzymes or proteins that emerge for this function then come to be seen as an intrinsic part of the new, extended,
spindle dynamics, made network by their own presence? Are these then the microtubule associated proteins (MAPs) we are
familiar with as part of tubule dynamics and what, then, if any, might their relation be to the centriole associated proteins
that form the core of the centriole and connect the angled microtubule planes?
The emergence of such
a protein would of course put the main centriole pair at risk and we can surmise that the electron-dense, i.e., ion rich,
pericentriolar material linking the mother and daughter realises some sort of protective function in this regard.
Obviously there is much to explore in
these structural dynamics and these are only small indication of the possibilities raised by recognising the quantum
mechanical principles of cellular self-organisation. We need to move on to the environment in which these take place.
> THE PHYSICAL CELL: FIELD
|