QUANTUM LIFE
Scientists need a manageable starting-point for their discussions, either in terms of
elementary constituents or in terms of a model of abstracted simplicity
John Polkinghorne, 1991
|
Quaternion = Julia Set = 'stressed' atom |
The approach of Quantum Aspects of Life to understanding living systems
and their possible dependence or otherwise on quantum phenomena, whilst admirably high level such as to allow or, indeed,
invite, discussion, does depend on the observation of a collective phenomenon and, as such, needs to incorporate bioelectromagnetics
research which covers both individual and collective sensitivities and behaviours.
When findings here are brought
to bear on the modern vision of living systems organisation, as we have seen in Adey's report, the essentially quantum
nature of living system emerges as a necessary requisite to bioelectromagnetic structuralism. In this much we can, therefore,
perhaps transcend the struggle of classical understanding to make the transition by examining the case when taken to logical
extreme, i.e., that living systems are the purest quantum systems we will ever have the privilege of examining. That
this might be the case was the implication of early sequence analysis using the mathematics of fractal geometry whereby the
~97% 'junk' DNA was found to have both structure and syntax and to in fact constitute a language whilst the ~3%
of the sequence data given over to biologically significant functionality was found to possess no such similar structure,
effectively then constituting 'noise' in an otherwise perfectly operating physical system. Indeed, Mandelbrot's
own Yale website points out that DNA is one of various phenomena that carry the signature of structured or 1/f noise.
|
Extract from Mandelbrot's Yale teaching website |
When this initial analysis was reported and the 'junk' material shown
to have all the structural traits of a language, it was proposed that they 'did not know what language that
might be or, therefore, what it might tell us' (Geake). Whilst then the idea that this language might be that of
quantum mechanics was not considered - and indeed for the mathematician the possibilities of there being one or more languages
makes the position complex - the quantum solution today is recognised as a required area of exploration
Indeed,
one can go further and posit that the properties that define living systems may derive solely from the quantum nature of their
foundation and evolution and that they are the extraordinary systems that they are because of their
emergence within and dependence upon the quantum nature of reality. We have, after all, only just begun to imagine
the possible technology of quantum systems and, therein, the form our quantum computers might have, what they might be made
of, how they might work, what they might look like and what they may be able to tell us. Given that all our imagining
is of so short a duration and still limited to the 'nuts and bolts' of the possible requirements, it is not so suprising
perhaps that at first glance we fail to appreciate the finesse that 3.8 bn years working is able to bring to organic quantum
systems self-organisation and, therein, 'natural' computing?
It is in this highly abstract light
that informed intuition and a priori reasoning tell us that if living systems are fundamentally quantum in nature
their self-organisation must accommodate the quantum nature of atoms and it is expected that at the
very least they need be:
- harmonic - resonant - and, therein, coherent systems
There is then also, embedded
within these properties being found, the possibility of extension to realise their being:
-
superconducting - supercomputing
We will develop
the argument for this being the case: the first three requirements and the grounds for living systems being capable
of supercomputing emerge by reworking the data of biological research and will be dealt with in the next section; the
general case for superconduction at physiological temperature is given below.
SUPERCONDUCTION IN LIVING SYSTEMS?
As Penrose
points out in his foreword to Quantum Aspects of Life (p. x) it does not require great sophistication to
achieve superconduction at room temperature: ' ... the very existence of high-temperature superconductors demonstrates
that collective quantum phenomena can take place with a relatively small amount of sophistication and without the necessity
of extreme cold.'
The case is simply illustrated by the graph below derived from data for all of the 'low'
and 'high' temperature superconductors currently known and depending on how we look at it, i.e., as linear
or power law relation, we can expect superconduction in atomic systems at temperatures in the physiological range -
~300 K - to be reached with anything between 8 - 18 atomic species involved.
At present
patent is being sought for a 195 K high temperature superconductor, so only 105 K to go!
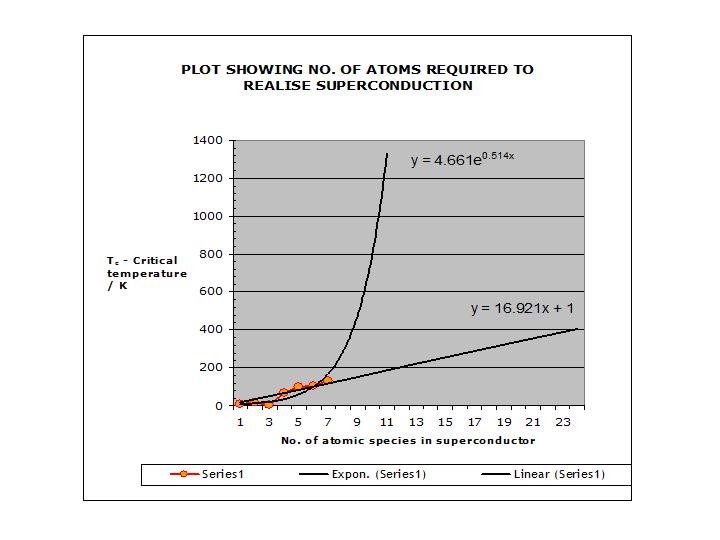
It
is very tempting to take the exponential function here as the 'natural' measure for sensitive systems modelling whereby
the linear accretion of atoms is transformed to non-linear response of the system, i.e., the whole is greater than
the sum of its parts, and, using the given figures, turns out as a particularly poetic function given that
it implies a relationship intrinsically bounded by the invariant measures of the Feigenbaum and e ...? Sheer
fortuosity or otherwise, it feels like that could be real ...
The possilbity of superconduction in living
systems has long been a subject of discussion, starting up almost as soon as the new quantum vision had undergone it's
first generation of within-discipline working and elaboration.
Miller (1991), exploring living systems foundation
from the perspective of understanding how animate-sentient behaviour might have traceable origin at the atomic level,
points out that 'It is certainly not widely recognised, even among physicists, that most of the small group who created
the principal constitution of terrestrial physics - quantum theory - regarded even stricly physical phenomena as having an
animate-sentient-like aspect! Other physicists agreed or at least found it possible'. Miller goes on
to reference most of the founding and influential community of early quantum theory development from Bohm, Bohr and Cochran,
through Dirac, Jeans and Pauli, to Weyl, Witmer and - of course - Schrodinger. In essence the approach was
founded on the logic that Jeans (1955) proposed whereby 'Organic life partakes then of the same freedom and spontaneity
that physicists have found at the root of material being.'
As Witmer (1966) explained 'These ideas
only seem strange when we apply them to micro-objects because, by habit and by virtue of our cultural environment, we think
of micro-objects as inanimate. It is no doubt true that to assert that micro-objects have volition is too strong a statement,
but ... they have attributes that are the vague beginnings of volition and self activity.'
Miller's paper initially proposes that the atomic
behaviours that will most readily display animate-sentient-like behaviours will be associated with momentum and energy - 'So,
for example, if energy is well defined, i.e., has vanishing indeterminacy, in a given situation, then the animate-sentient-like
aspect should be likewise well defined; whatever the effects [] they should be most demonstrable when energy interminacy
is smallest (cf. Jeans, 1943). Conversely, these effects should be least when energy interminacy is the greatest.'
These considerations then naturally extend to superconductivity where a macroscopically large portion of the electron population
occupies the same quantum-mechanical state; momentum indeterminacy and energy indeterminacy are vanishingly small in such
superconductivity states (SCS). From this, then 'systems in SCS should exhibit their animate-sentient-like aspects
to a high degree.'
Szent-Gyorgyi (1941) suggested that intracellular co-operation
and communication might be due to shared electronic energy levels, whilst London (1950) proposed that it might be the existence,
specifically, of SCS in biomolecules operating in STP conditions that 'might explain some of their unusual properties.'
'Little's (1964, 1965) calculations demonstrate the possibility of STP-SCS in organic polymers, while later calculations
indicate that thermal fluctuations probably destroy coherent macro-molecular order for quasi-one-dimensional systems,
they still allow the possibility of STP-SCS in micro-domains of 10-8-10-7 m (Little, 1967).
Noting that DNA is not sufficiently one-dimensional to be subject to Little's criteria for destruction of long-range order,
Ladik et al., (1969, 1981) presented scattering values for polycytosine valence and conduction bands as not excluding SCS
from DNA. The quantum field theory calculations of Del Giudice et al., (1988) leads "necessarily" to SCS in
cells. Halpern & Wolf (1972) also reason that biomolecular SCS may be restricted to randomly distributed micro-domains,
while the bulk of any sample would remain insulating or semiconductive due to high inter-molecular resistance in series with
the low intra-molecular resistance; they proposed the name "fractional" SCS.' (Miller, 1991).
Miller
goes on to list the various biological phenomena that have been proposed as being SCS-based: biological information
storage and processing; enzyme specificity and efficiency; carcinogenesis; cell growth; genetic coding; nerve conduction;
weak magnetic field detection and reproduction of quantum mechanical systems and concludes that 'There are, thus, theoretical
and empirical indications of SCS in biological systems. And, as already mentioned, systems in SCS should exhibit their
animate-sentient-like aspect to a high degree.'
From this Miller moves on to Frohlich's quantum mechanical
model of coherent biomolecular excitation and how 'he proposed vibratory modes for which energy is channelled into a single
mode when supplied at a rate in excess of a critical value, instead of being entirely converted into heat. This results
in an open steady state exhibiting long-range phase correlation. Frohlich considered this to be an example of Prigogine's
dissipative structures ... Most important ... the coupling involved leads to a drastic reduction in momentum and energy
indeterminacies, similar to that of Bose-Einstein condensation ... thus indicating that such systems should exhibit their
animate-sentient-like aspects to a high degree ... Generally, the considerable and growing empirical support demonstrates
non-thermal effects when organisms are irradiated with low-intensity microwaves. '
Miller's paper of over two decades ago indicates the vast
range of theory and experiment around the quantum foundations of the biological state and yet, of course, today we are still
having to persuade even science funding councils as to the need to understand living systems as quantum systems - systems
formed and maintained by radiation - in order to appreciate that they must of course be vulnerable to the electromagnetic
environment. Thus it is that we still have headlines about mobile phones and their 'possibly' damaging effects,
whilst in fact, for all those working at this level in living systems analysis, the question of damage or the 'influence'
of radiation is beyond doubt - indeed, a 'no-brainer' as they say today - and it is mechanism that most urgently
needs to be understood. One day - and soon perhaps - it will be recognised as quite 'unscientific' to claim
anything other.
OF CELLS AND CYCLOTRONS
|